Diet analysis
The InvaCost project is a research initiative that aims to estimate the economic costs associated with invasive species in Europe. Invasive species are non-native organisms that can cause harm to the environment, human health, and the economy. The project focuses on five key invasive species groups: aquatic plants, terrestrial plants, invertebrates, vertebrates, and fungi. The research is based on a collaborative effort between 27 European countries and involves the collection and analysis of data on the costs associated with invasive species in various sectors such as agriculture, forestry, fisheries, and health. The project also considers the indirect costs, such as the impact on tourism, biodiversity loss, and ecosystem services. The estimated costs of invasive species will provide policymakers and stakeholders with crucial information on the economic impacts of these species and the need for effective measures to prevent, control, and manage their spread. The project also aims to raise public awareness on the issue and promote cooperation among different sectors and countries to address the problem. Overall, the InvaCost project represents an important step towards a better understanding of the economic consequences of invasive species and the development of effective policies and strategies to mitigate their negative impacts.
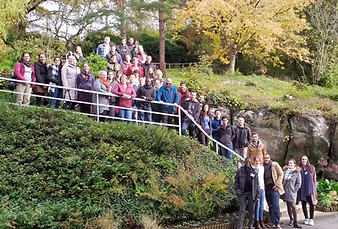
Diet analysis
Stomach content analyses can provide a direct insight into recently consumed items and, consequently if sufficient individuals were investigates, a species' feeding behaviour.
(A) The fullness index FI:
Following the approach of Hureau (1969), it can be expressed as the weight of ingested food (STW) in regard to the percental weight of the fish (FW) : FI= STW/FW. Only cardiac, cecum and pyloric part are usually considered without discarding contents of the intestine.
​
​(B) Feeding intensity
Based on the vacuity index (VI), the feeding intensity can be expressed as the percentage of empty stomachs in respect to stomachs that contained prey items.
​
(C) Diet analysis:
Personally, I prefer the estimation of F% (Frequency of Occurrence = number of stomachs containing each food item in relation to total number of full stomachs and N% (i.e. the number of individuals of each food item with respect to the total number of individuals). With these, the prominence value (PV) for each dietary component can be estimated following the approach of Hickley et al. (1994), indicating the importance of different prey items.
​(D) Diet overlap:
Using the prominence value, the diet-overlap index (a) between two species can be estimated according to the approach of Schoener (1970): with n being the number of food items, PVxi the prominence value of food item i in species xi, PVyi respectively the prominence values of food item i in species y. The estimated index varies between 0 (implying no overlap) and 1 when diets are identical (Wallace 1981).
(E) Dietary Niche Breadth:
Another regularly part of the analysis of fish diet is the computation of niche breadth. Generally, this is based on Levin’s index formula (Whittaker et al. 1973; Sa-Oliveira et al. 2013): where Bi is the standardized index of niche breadth; Pij the proportion of food category j in the diet of species I and n the number of food categories ingested by species i.
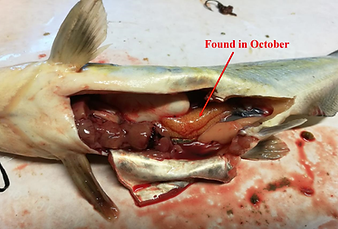
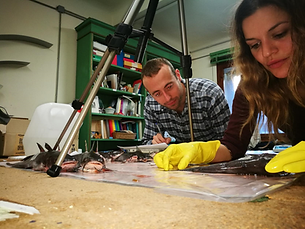
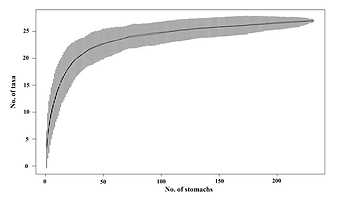
Stable isotope analysis
Stable isotopes analysis (SIA) provide long-term dietary information and allow a reconstruction of the studied communities’ trophic web and the investigation of niche partitioning (Boecklen et al., 2011; Layman et al., 2012). This is possible, because isotopic signatures relate with the trophic position (nitrogen, N) and with the main energy source (carbon, C) of a consumer (Layman et al., 2012). Additionally, SIA allows the estimation of trophic levels (Post, 2002) and quantification of feeding niches (Newsome et al., 2007; Jackson et al., 2011; Swanson et al., 2015). The degree of overlap among isotopic niches of different species can therein be assessed to evaluate the potential food competition between species (Layman et al., 2012; Balzani et al., 2016) or niche partitioning (Werner, 1979; Barbour et al., 2009).
​
(A) Trophic positioning
The trophic positions of a species (TPc) in a community can be calculated by applying the equation TPc= ((δ15Nc - δ15Nbase)/ΔN) +λ, where δ15Nc is the mean δ15N of the consumer, δ15Nbase is the mean δ15N of primary consumers, ΔNis considered as the standard enrichment of 3.4 ‰ between trophic levels and λis the basal trophic level (in many case = 1 for plants) (Post 2002; Britton et al 2018).
​
(B) Overlapping niches
To estimate and quantify intra-specific and community niche width, Layman metrics (Layman et al. 2007) can be calculated. With these, Layman described how the convex hull area occupied by a species in δ13C-δ15N isotopic space represents trophic diversity and can, therefore, be used as a quantitative indication of niche space. Jackson et al. (2011) extended these methods and strengthened their ability to cope with disparities in sample size by introducing the corrected standard ellipse areas (SEAc). With these, the degree of Stable Isotope niche overlap can be estimated and subsequently used as “a quantitative measure of dietary similarity among populations” (Jackson et al. 2012).
​
(C) Mixing Models
The application of dual plot graphs for δ15N versus δ13C of consumer tissues and food sources enables the determination of probable prey sources and combinations of prey contributing to the diet of predators (Phillips and Gregg 2003). Results from diet analyses can further be used to determine potential prey items for the application of Stable Isotope Mixing Models (SIMM) which indicate the relative proportion of a prey to a predatory trophic position. Results are generally presented as the average percent values with the possible range percentage for each prey item.
​
(D) Statistical inferrence
Several statistical approach can further be applies. For instance, a PERMANOVA can be performed to test for significant differences in δ15N and δ13C among species and to eventually determine significance in niche overlap between species.
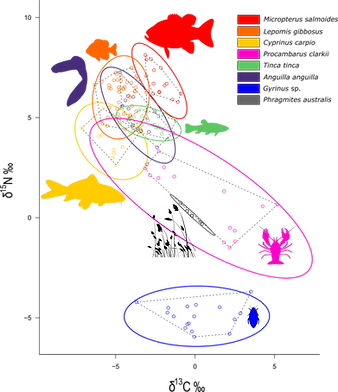

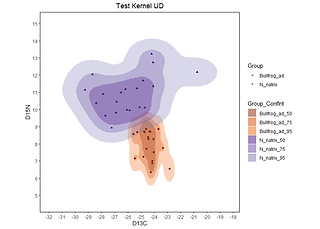
Long term data analysis
The analysis of long term biodiversity monitoring data is crucial to improve out understanding of locan and large scale biodiversity trends and hence, to expand our understand of biological invasions, ultimately helping us gain a comprehensive understanding of how ecosystems change over time. Having a long-term perspective is essential for effective management and conservation of biodiversity as it allows us to observe how species and ecosystems respond to various factors, such as climate change, habitat loss, - and especially - invasive species. Analyzing long-term data provides scientists with the ability to identify patterns and trends in species composition, abundance, and distribution. This information can be used to detect changes in ecosystems and to assess the effectiveness of conservation and management strategies. For example, long-term data analysis has helped scientists document the worldwide decline of amphibian populations and identify the causes of these declines.
Invasion biology is also heavily reliant on long-term data analysis. Invasive species can have significant impacts on ecosystems, including altering nutrient cycling, reducing biodiversity, and changing ecosystem processes. By monitoring invasive species over time, scientists can assess the success of control measures and predict the long-term impacts of invasive species on ecosystems.
​
My research focuses on a mixture of the study of interactions between native and non-native species over time, as well as drivers faciliating the success of non-native species introductions.
​

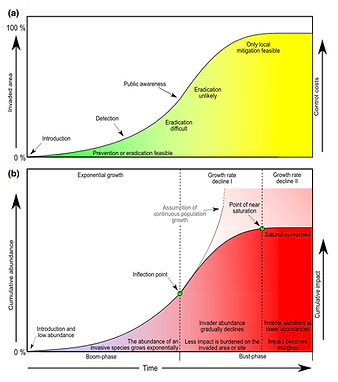

Study sites
Lake Arreo, Spain
Lake Caicedo Yuso-Arreo, further on referred to as Lake Arreo, is a peculiar ecosystem in the Iberian context, as it is one of the eight Iberian lakes on a saline chimney (Camacho et al. 2009). In addition, it is the only natural lake in Basque country. In the 19th century, its fish community was composed of trout (Salmo trutta Linnaeus, 1758), European eel (Anguilla anguilla Linnaeus, 1758) and tench (Tinca tinca Linnaeus, 1758) (Madoz, 1845). At the beginning of the 21st century however, trout was already absent and alien species like largemouth bass (Micropterus salmoides Lacépède, 1802) and pumpkinseed (Lepomis gibbosus Linnaeus, 1758) were detected in surveys under the Water Framework Directive ecological status monitoring network (Rico 2004). The fish census carried out in 2010 (Monteoliva et al., 2011b) detected common carp (Cyprinus carpio Linnaeus,1758) and quantified dense populations of invasive Largemouth bass Micropterus salmoides and Pumpkinseed Lepomis gibbosus.
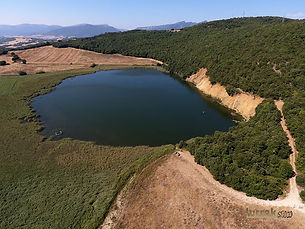
Arno River, Italy
In Tuscany, allodiversity is very prominent, making up more than 3% (48 species) of freshwater fauna, with the Arno River basin presenting the highest amount of non-indigenous species (NIS) (Nocita 2007; Tricarico et al. 2008). Due to the high anthropogenic influence, the Arno River’s environment, biodiversity and species composition were highly affected. Nocita (2007) and Nocita et al. (2010) showed the presence of several invasive species with diverse invasion histories and low abundances and even the loss of several native species within the inner Florence city section. Such exerted ecological impacts, that can be defined as a measurable change of variables in an ecosystem over time, strongly increase during first stages of the establishment according to population growth, eventually reaching a plateau phase according to demography or predator-prey dynamics, adaptation or interactions among species and lastly, depending on varying geographic scales (Ricciardi 2003; Strayer et al. 2006) - which is apparently the case for various non-native fish species in the Arno River.
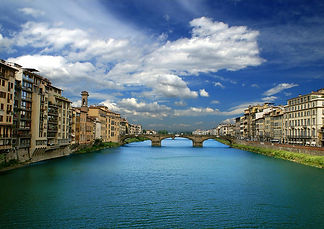